
Is life on Venus possible? Piotr Stankiewicz talks to Janusz Pętkowski from the Massachusetts Institute of Technology – a member of the team who, in September 2020, declared life on Venus entirely feasible. Finding extra-terrestrial life beyond planet Earth is one of the greatest dreams of many scientists. Last year’s discovery about Venus created a furore, but we still have to wait for the final verdict. This is a good moment to consider how life might function on a completely different planet to Earth and whether chemistry holds the answer to the Fermi paradox.
Piotr Stankiewicz: Among other things, I am an astronomer by education, so I understand that the rhetoric of scientific discovery is different to that of the tabloid press. That’s why I am not starting with a question about whether there is life on Venus. I will start with the facts. From observations of the Venus spectrum, we know that its atmosphere contains phosphine, which is a potential sign of life.
Janusz Pętkowski: Phosphine is the name of the chemical compound, PH3. One atom of phosphorous, plus three atoms of hydrogen. In short, it seems that phosphine is present in Venus’s atmosphere although, of course, as with every scientific discovery, further observations will be necessary in order to confirm our thesis. If phosphine really is present in the clouds of this planet, we have absolutely no idea where it has come from. Either some sort of geophysical or photochemical process – unknown to science – takes place there, or it is a biochemical process. The latter possibility would mean that some form of life exists on Venus.
Let’s go deeper. How does the presence of phosphine on Venus indicate a sign of life?
Above all, we should ask which gases present in the atmosphere of the planet might indicate the existence of life. Although the question itself is simple enough, it is difficult to answer, because we don’t know upfront what biochemistry extra-terrestrial life will have. We began by creating a database in order to determine which gases could be interesting from this perspective. Of course, we are talking about thousands of chemical compounds, and phosphine is just one of them. What is interesting in this case, is that phosphine has appeared in the atmosphere of a rocky planet…
Let’s spell it out: in the solar system, the rocky planets are Mercury, Venus, Earth and Mars, while Jupiter, Saturn, Uranus and Neptune are gas giants.
On the gas giants, phosphine can be created naturally; in the deep layers of the atmosphere, where the temperatures and pressure are very high and there is abundant hydrogen. However, we don’t really know what processes, other than biochemical ones, might explain its creation on the rocky planets.
Have you analysed all the processes under which it can be created?
Yes. One pathway for the production of phosphine is through atmospheric discharges. In order to achieve the reduction of phosphorus – in this case, to combine it with hydrogen – you need to supply energy. This can happen, for example, during a lightning strike. These can actually happen on Venus – they were studied by the Soviet Venera programme’s VeGa probes. But in order for as much phosphine as we observed to be created, those atmospheric discharges must have been several orders of magnitude greater than those on Earth. And that is not very likely.
It is not just about creating that phosphine once, but also compensating for the ongoing losses. Solar radiation will continually be breaking it down. Phosphine also takes part in various reactions. In short, if we can observe phosphine on Venus, this means that there must be some process going on there that causes it to be produced continually. Atmospheric discharges cannot secure this supply. We have also ruled out reactions between phosphorus oxides and other compounds, the delivery of metal phosphides by meteorites falling on Venus, and many other processes. All this indicates that phosphide could not be created in sufficient volume to compensate for its continual losses. There is way too little from this. Quite simply, the numbers don’t add up.
So, what then? A process completely unknown to science could be behind the presence of phosphide in the atmosphere. If so, I would suggest some strange, multi-phase, photo-chemical process. But there is also a hypothesis – and it is only a hypothesis! – that it could also be a biochemical process, i.e., connected with life. And that is not a hypothesis, nomen omen, from outer space. It comes from what we know about life here, on Earth. We know that life has sufficient chemical power to create phosphide from phosphorous oxide.
If there is life on Venus, what might it look like? I ask, because the conditions on that planet are rather hellish. As a reminder: the average surface temperature is 470°C and the pressure is over 90 (Earth) atmospheres.
The surface of the planet is too hot, so we’re talking here about life in the atmosphere, in particular within the Venusian clouds, at a height of between 50 and 60 kilometres, where the temperature and pressure are significantly lower. And, of course, we are only talking about micro-organisms and not ‘living balloons’, as Carl Sagan once proposed.
Sagan was probably thinking of Jupiter, but never mind. How do we know that these ‘living balloons’, or, for example, birds, are not feasible?
There are no traces of evidence to suggest that complex, macroscopic life, which is capable of independent movement, exists on Venus. Moreover, it is hard to imagine how it would function without contact with the surface of the planet. One can only consider a biosphere like this for Venus. If, indeed, any sort of biosphere exists there.
Look how it works down here. There is, of course, a huge amount of life in the Earth’s atmosphere, but it’s not true to say that the micro-organisms that live in the air don’t need the surface of the planet and can have a life cycle independent of it. In the case of Venus, we are considering life that has no contact whatsoever with the surface of the planet. That is very difficult.
Let’s start from the purely physical problems: such life-forms would have to be fighting against gravity all the time – how would they avoid falling too low, down into the too hot layers of the atmosphere? Next, one could assume it lives on a droplet of some sort. But droplets in the atmosphere are subject to various physical changes; now vaporizing, now condensing. When they connect, they become heavier and fall down to levels where it is too hot. However, from the chemical perspective, life on Earth uses metals to catalyse proteins. Yet metal compounds are generally earth-bound substances. So how would they get up into the atmosphere? How would the right amount be captured? Are we talking here about life without metals? Is that even possible?
One thing is certain: if there is life on Venus, it is different to that on Earth. But what it’s like, precisely – that, of course, we don’t know.
Where could it have come from?
The emergence of life and the survival of life are two different things, of which the first is significantly more difficult. Once life has emerged, it can adapt very well to its new environment. The emergence of life is in a different league altogether. The majority of well-known theories assume that it takes place in liquid – on Earth, in water, of course. In an atmosphere, which is a gassy environment, it is going to be harder. Therefore, it is of course possible that life emerged straight away up in the Venusian clouds, but it is not very plausible.
A second option is panspermia – the export of life from Earth on meteorites. Then we would be talking about life forms that came from Earth, but have evolved into something different. A third scenario is that life emerged on the surface of Venus and only later ‘migrated’ up into the clouds.
But could life have emerged in those surface conditions? The temperature there is higher than in a sterilizer or an oven.
That is what it is like today. Here, we should remember the model proposed by Michael Way. Under this model, the surface temperature of Venus was around 30°C for many billions of years and the surface of the planet had liquid water – oceans, lakes, great bodies of water. According to this theory, this is how it could still have been some 700 million years ago.
What happened next?
We don’t precisely know. Some radical change occurred or, more likely, a catastrophe, presumably a sudden increase in volcanic activity. One can visualize it: all the volcanoes on the planet erupt at once. This caused the majority of Venus’s crust to melt, releasing vast quantities of CO2 into the atmosphere, which was not reabsorbed with sufficiently speed; the greenhouse effect took hold and …
…and now we have the Venus of today.
Yes. And now, moving onto the next level of speculation, look at how this is all links together so interestingly. One can imagine a scenario whereby, once upon a time, there was life on the surface of Venus in those waters and oceans. Only later did the radical climate change occur. Note: radical, but how violent? In the lifespan of a planet, this can be a sudden change, but in relative terms this could take millions of years. And, if it was slow enough…
“… Life, uh, finds a way,” as Ian Malcolm says in Jurassic Park.
Escaping the surface of a planet that had become uninhabitable, life could have moved into the upper part of the atmosphere, where the conditions were more hospitable. If this change was not too sudden, it could simply have happened through evolutionary adaptation.
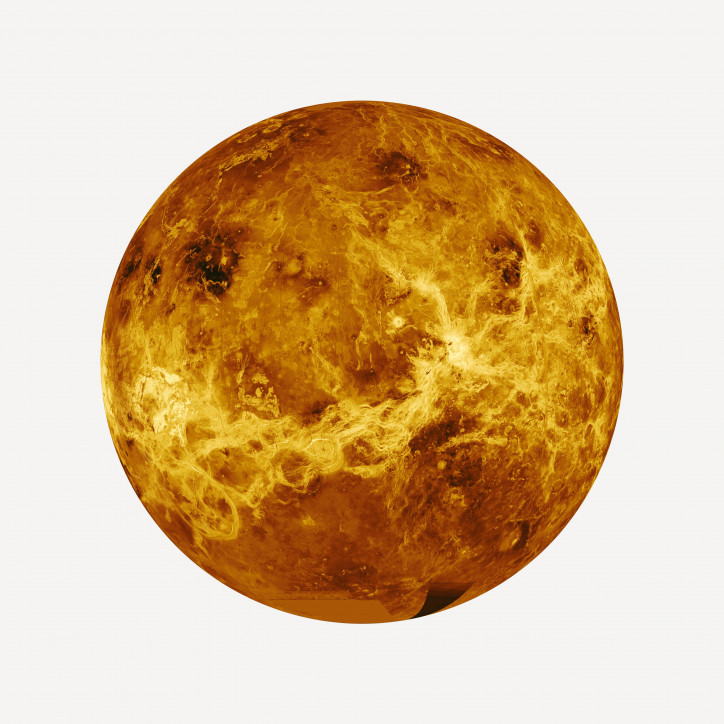
You are a scientist, so I won’t force you into further levels of speculation, but it’s difficult for me at this moment not to think that maybe 700 million years ago there could have been intelligent life on Venus which, by its own hand (or tentacles) caused the catastrophe and ‘destroyed’ the whole planet. Maybe we are talking science fiction, but not entirely. In the era of Greta Thunberg, this is not a ridiculous idea. Maybe Venus is a sort of older sister of Earth, and the fate of life on Venus is not our past, but our future?
An interesting line of thought, but completely beyond the remit of science. That’s not just another level, but the ninth circle of speculation. At this time, we have no signs in the heavens, or on Earth, or from Venus that could suggest something like this.
But since we are speculating, it’s worth adding one more thing. The Venusian clouds contain almost no water, but instead there is a lot of sulphuric acid. Theoretically, we could imagine a life form that didn’t use water as a solute, but H2SO4. How would life like this get started? In acid, it is much harder to build the basic chemical ‘blocks’ that life depends upon. Once you have them, you can start to think about building biochemistry. Only that, in acid, you don’t have anywhere to take them from. This is the great riddle: how life like this would start.
Let’s take it further. Maybe life got going in an aquatic environment on Venus, but later transformed itself and adapted to sulphuric acid? This raises another interesting question. Is life that forms in water able to change its own biochemistry under evolutionary pressure and ‘jump’ from H2O to H2SO4? Is life able to perform such a radical change by itself? This is a question in part for a biologist and in part for a philosopher.
That’s why the two of us can have such a great discussion! We can also imagine, in the same way, that humans – consciously intervening in their own development – will radically change, maybe into something that will no longer be human. If humanity can one day record consciousness onto the internet, it will no longer be dependent upon that weak construct of carbon and water, as consciousness is today. It will be a real change of medium.
Only, the difference between that post-human internet copy of 2220 and us here in 2020, is that there will no longer be any strict biological evolutionary connection between us.
Agreed. This post-human will be our descendent but more on a ‘spiritual’ or ‘project’ level than on a biological level. This is where I would end our philosophical diversion. Let’s get back to the science, but no longer on Venus. Let’s talk about the possibilities of extra-terrestrial life in general.
We can go through it point by point. There are five main conditions that must be fulfilled to talk about life. The most general truth is that life is based on the transfer of information.
That’s clear. ‘Like gives birth to like’. Without information there is no inheritance, no continuity.
Life on Earth stores this information on carbon polymers. Discussions about ‘extra-terrestrial life’ generally start from analysis of whether there could be a biochemistry based on the polymers of some other element. We have several candidates other than carbon: silicon, boron, sulphur and, possibly, germanium.
Why those ones specifically?
Because they are able to create polymers – that is, very long chains of atoms. In practice, we can rule out germanium because it doesn’t make long enough polymers. Moreover, it can’t have only one. Branching must be possible. For this reason, sulphur can also be excluded, as it doesn’t branch well. In contrast, boron branches too much. Everything interconnects and you end up with a ‘thicket’ of boron with no diversity. And so, we are left just with carbon and silicon. Carbon is obvious, because the whole of life on Earth is based on it. That’s why the majority of the speculation – scientific and beyond – about other non-carbon forms of life is around silicon. But silicon also has its faults.
What faults?
It has a very high affinity with oxygen, so it really likes to bond with it. Bond for good and, literally, into concrete, because it forms silica (SiO2), otherwise known simply as sand. The bond between oxygen and silicon is so strong that it cannot be broken… and that is why rocks are so stable.
Now we can move on to the second condition that must be fulfilled for us to talk of life. This is the very ‘scaffolding’ of carbon or, hypothetically, of silicon, which generally takes no part in chemical reactions. Life needs a chemical construction like this not only to provide stability, but also reactivity.
Which leads to the golden thought that life is not just about survival, but also about action.
This makes a lot of sense on the chemical level. Life is a self-regulating process. It needs stable elements, but it must also tear those bonds apart. For this, ‘functional’ elements are necessary: for life here on Earth, these are oxygen, sulphur, nitrogen or phosphorous. Carbon bonds well with them all and this is where it has an advantage over silicon.
There could be an alternative biochemistry of life based on silicon, but electronics is already built on it. What is so special about silicon that we can talk about it in both contexts? Is there a deeper physical-chemical reason for this, or is it just coincidence?
More of a coincidence.
Let’s get back to the subject then. What is the third condition for life? Am I right in thinking it’s temperature?
The temperature cannot be too high, as the complex chemical bonds on which life depends will come apart. It also cannot be too low because then nothing, chemically, can happen. But there isn’t a single, defined temperature range in which we look for life. Because this is not so much about the temperature itself, but rather the relationship between temperature and reactivity.
Look at it this way. In the temperature of liquid nitrogen, life on Earth is dead. Simply put, none of the reactions that are necessary for life will take place there. But if we take elements that don’t create stable chemical bonds at room temperature and chill them right down, then it may turn out that life works perfectly. Again, silicone is a good example. It is highly reactive, but we can reduce that reactivity if we lower the temperature.
All this is very tightly connected to the fourth condition: life needs a solvent. All of life on Earth uses water for this purpose. Could a different form of biochemistry use something else?
That’s a question for you.
We don’t know anything for certain. I remind you, that life other than life on Earth is still a hypothesis. Many substances are being analysed in this respect; not only ammonia or sulphuric acid, which we referred to in the case of Venus, but also cryogenic liquids such as liquid methane or liquid nitrogen, which take the temperature range down.
And up?
Well, not really! They say, perversely, that ‘water is a hot solvent’. Of the known solvents that life could make use of, water is one of the hottest. That’s why life on Earth is rather a high temperature life – there’s not much room to get hotter. Theoretically, there is the possibility that liquid sulphur…
In that case: hell! Checkmate to the atheists!
I don’t know how much there is of it in hell, but liquid sulphur has a temperature of 115–445°C (at atmospheric pressure). I think that is already rather too hot. Complex chemical compounds are liable to break down. Let’s look at it another way. We have super-cold liquids, which we know are present on the moons of the gas giants. Titan definitely has liquid methane and it is possible also that Triton has liquid nitrogen. It is certainly worth analysing. With the proviso that we also have a fifth condition: solubility.
In order for life to exist, our chemical compounds must be able to dissolve in our chosen solvent, and not become a precipitate. One of the miracles of life is how even chemically-large compounds, like DNA or proteins, are always found in a solution. But solubility is regulated by temperature. In general, the lower the temperature, the lower the solubility. It is this, in my opinion, that is a serious impediment to the possibility that life could exist in such low temperatures. At a temperature of –200°C, the solubility is simply going to be horrendously low. We don’t really know how to get around this.
To finish, let’s go back to the world of speculation. Let’s assume that somewhere life has evolved, for example, in liquid methane. Let’s imagine that it is a macroscopic form of life; some kind of animal, extra-terrestrial fish, or other freakish thing. Do we have the right to assume that, due to the slower chemical processes at lower temperatures, this life form will function more slowly, in the most basic sense of the word – i.e., move, think and evolve more slowly?
That’s the million-dollar question. Remember that, as I like to emphasize, ‘you adapt your chemistry to your solvent’. Life in liquid methane will be based on chemical compounds which, in ‘that’ temperature will be just as reactive as ‘our’ compounds in ‘our’ temperatures. Science fiction enjoys imagining that life in a cryogenic ocean goes more slowly than on Earth but, in reality, we have too little data to draw any conclusions about this.
Because, you see, in the meantime I have joined the dots and solved the Fermi paradox; the silence of the cosmos. Why is it that we have still not made contact with an extra-terrestrial civilization? This is my thinking: if water is a high temperature solvent, and the speed of reaction is dependent upon temperature, this means that life on Earth is one of the ‘fastest’ in the universe. However, other biospheres – and civilizations – may look so slow from our perspective that they appear almost dead. That is why the universe is silent – because the aliens operate in different, much slower timescales.
That’s a very interesting interpretation but, as I said earlier, there is no basis for drawing such far-reaching conclusions. From the scientific perspective it looks like this: life requires all five conditions to be fulfilled. We must have a suitable polymer, and suitable reactivity, temperature, solvent and solubility. It may be the case that we still don’t have hard evidence for extra-terrestrial life, quite simply because it is not so easy to combine these factors. This resolves the Fermi paradox just as well as your extravagant thought experiment. And you know yourself how science works: if two hypotheses do the same work, we always choose the simpler one.
Parts of this interview have been edited and condensed for clarity and brevity.
Janusz Pętkowski:
An astrobiologist. He is searching for extra-terrestrial life and the answer to the question of whether biological laws are universal. He works at the Massachusetts Institute of Technology (MIT) and is one of the founders of the Polish Astrobiology Association.