
Two biologists, father and son, both bearing the same name, share with our readers some highly interesting hypotheses regarding the beginning of life on Earth.
Around 4.5 billion years ago, on the periphery of the Milky Way, a cloud of molecules rotating around a small star thickened into bits of matter, which were tiny amid the immensity of the stars. Soon one of these bits of matter started to behave strangely; an atmosphere appeared above its surface and on the surface itself water, which contained complicated chemical compounds. Finally, in addition to the blue of the sky and white of the clouds, the planet was decorated in a strange green colour, while the thin atmosphere wrapping the globe became saturated with a poisonous gas: oxygen.
These were the consequences of the emergence of life on Earth. Where did it come from? Let’s go back in time to the very beginning. What follows is a family chronicle of all living beings.
The roots of the family tree
In Charles Darwin’s notes, we find a hand-made sketch presenting a relationship diagram of all living organisms: a ‘tree of life’ modelled on a ‘family tree’. Interestingly, Darwin himself did not in any way speculate about the beginnings of life and wrote nearly nothing about it; he believed that we will never find out how life was created, yet he was interested in knowing how it had evolved from simpler forms to millions of species, including humans. Darwin was not the first person who figured that all species originated from one common ancestor. The same idea came to the mind of George-Louis Leclerc, better known as Buffon, in the 18th century, and many others later on. It really wasn’t until the second half of the 20th century that modern genetics supplied proof for this.
All living organisms, from bacteria to humans, have genes recorded on long strands of a chemical substance known to all as the three-letter abbreviation DNA. For the past few decades, we have been able to read off the text written in the DNA (although understanding what we’ve read is a different matter altogether; we’re still having issues with that). It seems that genes tell the story of our past and indicate the existence of a common ancestor of people and other primates; if we go back even further in time, we find the protoplast of all mammals; further yet, the ancestor of all vertebrates; finally, the eukaryotes, or singled-celled and multicellular organisms (including plants) that have a complex cell structure.
But as we go further and further back, to around two billion years ago, we start to lose the trail, somewhere between the bacteria, eukaryotes and archaea (which are much like bacteria, but also possess many characteristics close to ours). We can only say for sure that at the root of the tree, we have microscopic single-celled organisms that probably formed the beginnings of all earthly life.
If we speak of the history of life on Earth, the newer it is, the more we know about it. The last half billion years, dominated by large multi-cellular beings, often possessing an endoskeleton or exoskeleton, are superbly documented in fossils. These types of traces are a dime a dozen. Some of them are so well preserved that we can study their DNA or proteins; the oldest protein ever studied by scientists is the collagen preserved in the skeleton of a tyrannosaurus from 68 million years ago. This may seem like an unimaginably long time for us, yet from the perspective of the history of the planet, it isn’t much. In the times of the tyrannosaurus, the fossilized skeletons of another giant dinosaur, the stegosaurus, were already over 70 million years old.
The rock speaks
The oldest microfossils, or the remains of microorganism structures, are no older than one billion years. Yet we also have traces of life of other types, which are surprisingly plentiful if we look well enough. And they are amazingly old.
Proof that life began not millions but billions of years before the earliest fossils, is contained in so-called banded iron formations. These formations were witness to an important event in the history of the planet – the Great Oxidation Event (also called the Oxygen Catastrophe); the first documented ecological calamity on Earth. Banded iron formations, as the name itself suggests, have a characteristic pattern consisting of red bands, which get their colour from oxidized iron (the same compound that gives rust its colour). There was plenty of iron in the oceans of the young Earth, yet its deposits were blue in colour. They wouldn’t become red until molecular oxygen, or O2, appeared in the atmosphere. As long as the oxygen content in the air wasn’t too high and remained subject to seasonal fluctuation, deposits containing iron would alternate between a bluish and rusty colour. After some time, there was so much O2 in the atmosphere that all the deposits took on a uniform red colour.
The molecular oxygen that we need to breathe is very active, and there is only one source of it on Earth: live organisms that are capable of photosynthesis, namely plants and bacteria. The presence of red deposits containing iron oxide is indisputable proof for the existence of vibrant life nearly three billion years ago.
But why do we actually speak of a ‘Great Oxidation Event’ or even ‘Catastrophe’? This is because we suspect that the oxygen-rich atmosphere led to the greatest extinction of anaerobic microorganisms in history, as highly reactive oxygen was poisonous for them. What’s more, the change in atmospheric composition (mainly a drop in the concentration of carbon dioxide) 2.5 billion years ago evoked the glaciation of the entire planet, which lasted 300 million years.
Isotope studies also provide evidence for the existence of life on Earth. Isotopes are different forms of the same chemical elements. Although we usually associate them with radioactivity, many isotopes do not show any signs of it. For example, carbon naturally occurs in two isotopes, 12C and 13C [we still have 14C, but it decays rather quickly and cannot be used in studies reaching deeper into the past than 50,000 years – ed. note]. Carbon 13C is a bit heavier than 12C and as a result, there is relatively less of it in living organisms (which readily take in carbon from the air as carbon dioxide). Based on the proportion of 12C to 13C, we can determine if our finding was once alive or not.
Nearly every year, we see new publications presenting ages-old record-breaking fossils, the isotope content of which prove that they indeed constitute the first traces of life. Three years ago, Nature ran an article that described structures resembling layered microbial mats (stromatolites) that were 3.8 billion years old. To this day, the content of the article is still the subject of intense dispute, but everything seems to indicate that life on Earth has been in existence for at least 3.5 billion years. Therefore, life appeared on the planet relatively quickly once it had formed and cooled down, although it was limited to single-cell organisms for over 80% of its history, as large multicellular plants and animals are a rather new finding.
Isotope analysis is providing us with an increasingly fuller picture of the world of the first microorganisms. With the help of sophisticated methods, we were able to determine not too long ago that the microfossils which are 3.5 billion years old were indeed once living organisms, and were also capable of photosynthesis as well as producing methane.
Life from a test tube
But neither genetics nor the chemical analysis of rocks and fossils will provide us with an answer to the question of how the first cells were created from simple non-organic compounds such as carbon dioxide, water and silica; here we speak of aggregates of complicated molecules that are complex, capable of self-healing and evolution, and have difficult to pronounce names, such as deoxyribonucleic acid or nicotinamide adenine dinucleotide.
And here’s the bad news: it’s almost certain that we will never find out for sure where life came from in the first place. But we can try to discover how it could have been born. We know that two fundamental steps are needed to create life from non-organic matter. First of all, organic compounds such as sugars, amino acids and nucleotides had to appear. Second, a biological evolution had to be triggered, or the creation of structures that were capable of replication and evolution. How did that happen? To this end, we can formulate hypotheses, which can then be tested in the laboratory.
That’s what happened to the hypothesis put forward in the 1920s independently by two scientists, J.B.S. Haldane and Alexander Oparin. Both of them suggested that organic compounds can be spontaneously created from non-organic compounds. The statement was outrageous at the time; only a few decades earlier, the general belief was that organic compounds differed in principle from non-organic compounds and many even suspected the existence of a specific life force, a vis vitalis, present only in the first type.
However, both Oparin and Haldane were right, after all. 30 years later, Stanley Miller (together with his promoter Harold Urey) showed that complex organic compounds – sugars and amino acids, or the basic components of proteins – do indeed appear in a mixture of water, ammonia, hydrogen and methane, through which electric sparks pass. A few years ago, after Miller’s death, some of his samples were analysed using modern mass spectroscopy methods, and even more different organic compounds were discovered. Although the mixture of gases used by Miller did not really resemble the primary atmosphere of the Earth, a number of similar experiments in a variety of conditions have been conducted since that time, achieving a spontaneous synthesis of not only sugars and amino acids, but also nucleotides – the building blocks of DNA and RNA. Two years ago, a group of scientists from Glasgow demonstrated that nucleotides can spontaneously compound into polymers resembling DNA.
Not necessarily oxygen
Each organism is made up of complicated chemical compounds. To live, it needs energy, which allows the creation and maintenance of these compounds and drives a variety of processes: movement, collection of substrates from the environment, growth and multiplication. Life on Earth is based exclusively on one method of energy conversion, this being the chemical reaction of the oxidation of certain substrates by others, which in turn become ‘deoxidized’ – something professionally referred to as ‘reduction’. If we mix ‘deoxidized’ sulphur and ‘deoxidized’ carbon with an oxidant – in this case saltpetre – the mix, commonly referred to as gunpowder, will easily undergo a sudden reaction of the reoxidation of sulphur and carbon, releasing lots of energy at the same time. The cannon will fire. Our organisms are constantly producing energy in a similar manner.
For the majority of contemporary organisms, the oxidant that allows for this energetic supply of all processes is oxygen in the atmosphere (or dissolved in water). Yet oxygen did not appear until life ‘learned’ how to use an unlimited source of energy: solar radiation. With its help, carbon dioxide from the air undergoes reduction, producing a variety of molecules and releasing oxygen in the process.
While trying to solve the puzzle of the beginnings of life, we need to realize the fact that oxygen does not necessarily need to be the oxidant. After all, in gunpowder the oxidant is saltpetre, while many contemporary microorganisms make do with other chemical substrates that enable the process of oxidation and reduction. It could have worked the same way at the dawn of life on Earth.
Oparin and Haldane assumed that the source of energy powering the synthesis of organic compounds were electric discharges in the atmosphere (Miller’s experiment confirmed such an option), but did they occur frequently enough? Perhaps there were other sources of energy in the form of other oxidants?
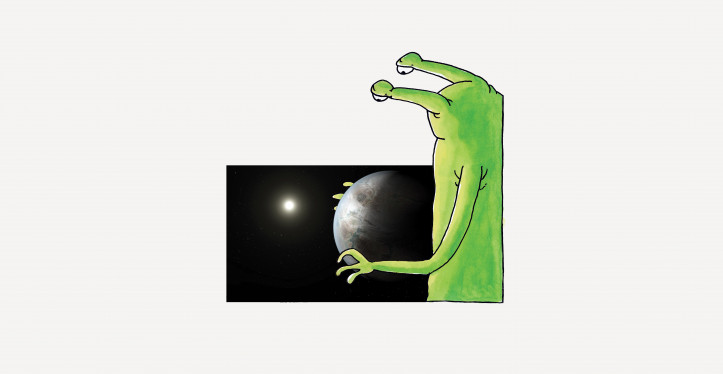
From the depths or the surface?
In the 1980s, hot deep-sea springs were discovered, namely hydrothermal vents graphically referred to as ‘black smokers’. Water under great pressure at two kilometres below the surface of the sea, heated by lava to several hundred degrees, penetrates the rock, washing out enormous amounts of non-organic compounds of carbon, sulphur and iron, including lots of methane and ammonia, which there wasn’t much of in the primary atmosphere of the Earth, contrary to what Oparin and Haldane had imagined. Life flourishes around the vents, driven not by energy from the sun, but by the chemical compounds supplied with hot water. The discovery of hydrothermal springs gave rise to hypotheses which suggested that the energetic potential needed to supply life processes can be produced thanks to reduced sulphur and iron compounds, as well as reduced carbon or methane, coming out from the depths of the Earth.
There is at least one additional possibility: hydrothermal springs on the surface of dry lands. Although the geochemistry associated with such springs is different, they are also capable of supplying enormous amounts of various more or less complicated chemical compounds that microorganisms can use as a source of energy and as building blocks; but in addition, they are also subject to sunlight and ultraviolet radiation (UV). And that could have been key. As was proven a few years ago, thanks to UV rays, RNA synthesis can occur based on a simple chemical compound: hydrogen cyanide. John D. Sutherland, whose team made this discovery, described reactions that could have contributed to the spontaneous creation of not only RNA, but also amino acids and fats from hydrogen cyanide and a few other simple compounds.
We can therefore confidently assume that in the conditions prevailing on the young Earth, spontaneous synthesis of organic compounds, perhaps even rather complex ones, did occur. But that only explains the first part of the puzzle. We still need to find the answer to the question of how evolving organisms could have been created from these compounds. A key matter here is the ability to evolve, therefore the ability to self-replicate with errors (mutations) that make the creation of new forms possible. We actually know of only one system in nature that can do that: DNA. There is a small issue though; for DNA to replicate, you need protein.
The magic molecules
In all living organisms, genes are written on the strands of DNA, and coded in the genes of all organisms are proteins, which are both the basic building block of our cells as well as the main element of the cell machinery; proteins build other proteins and all other components of our cells, synthesize DNA and perform complicated chemical reactions that keep us alive by supplying energy. Life is protein, and just as there are no proteins without DNA, there is no DNA without proteins, or enzymes that replicate DNA during cell division.
The information contained in the DNA is needed for protein synthesis, and protein is needed for DNA synthesis. Thus yet another serious issue concerning the creation of life appears: what came first, the chicken or the egg? DNA or protein? We happen to know the answer to the first question, because reptiles, amphibians and fish were already laying eggs millions of years before birds appeared. The second issue is much more difficult. We can presume that, quite exceptionally, the truth indeed lies somewhere in the middle. Quite literally in the middle, actually: there is one more chemical substance on the road between the information coded in the DNA and the protein. Before the protein is created according to the recipe, or a gene written in the DNA, that recipe first needs to be written on a ‘scratch pad’. It’s as if we were to take a cookbook, choose an excerpt on a specific dish and copy it onto a piece of paper that we could conveniently stick on the refrigerator or somewhere above the counter. Proteins are not built based on DNA directly, but rather on the basis of that draft, which is written on the single strands of a substance known as RNA.
One of the most incredible (and Nobel-awarded) discoveries in the history of molecular biology was the conclusion that RNA is not passive like DNA, but that it can take on the role of an enzyme called ribozyme. Some of these ribozymes are able to synthesize RNA, albeit to a limited extent. Therefore, RNA can simultaneously be an enzyme and an information medium. Scientists succeeded at creating such RNA particles in the laboratory. Not only were these particles able (to a large degree) to self-replicate, but as they did so with errors, they would evolve, improving their performance in a short time.
All of this seems to suggest that the first evolving molecules (since it’s hard to speak of organisms just yet) could have been complex ribozymes; it is the remainders of these ribozymes – or should we say, molecular fossils – that we all carry with us in our bodies.
Cosmic hypothesis
Yet we cannot rule out that life was created outside Earth. We know today that certain bacteria are able to survive in extreme conditions that would otherwise be fatal for other living organisms, so maybe some bacteria made it to our planet along with an out-of-system asteroid, like stowaways. This is rather unlikely though, as asteroids originating beyond our solar system are an extremely rare phenomenon, while the bacteria stuck to it would need to spend hundreds of thousands (if not millions) of years in transit. Besides, even if that was what happened, it only explains how life ended up on Earth, pushing the problem of the creation of life to another planet. Nevertheless, we could try to test such a hypothesis. Experiments are being conducted to check the resistance of bacteria to extreme temperatures and it seems that certain bacteria, hidden deep in rock, indeed managed to survive the impact of a meteor on Earth. They are also able to survive in outer space, as long as they are protected from cosmic rays.
Then we have some ideas that are really astounding. One of the discoverers of DNA, the Nobel prize winner Francis Crick, once put forth a thesis that life had been intentionally disseminated by a higher civilization. Yet this vision rather proves the eccentricity of Nobel prize winners and suggests that we should not unconditionally accept everything they say. It’s really not a matter of whether it’s probable or not, but rather that the idea is entirely non-scientific, meaning that there is no way of experimentally testing such a hypothesis. On a side note, Stanisław Lem himself made fun of such a scenario by suggesting in one of the Ijon Tichy stories that life on Earth had its beginnings in a booze-laced prank of a certain Bann and a certain Pugg, who, having spilled a nasty mix of gelatine and amino acids, started to stir it using a coal shovel bent to the left, which would explain the left-handed amino acids that build protein.
Translated by Mark Ordon