
Dr Paweł Preś, an astronomer at the University of Wrocław, talks about the importance of keeping a close eye on our closest star.
Jan Pelczar: Why do you never let the sun out of your sight?
Paweł Preś: A scientist is constantly on the front line.
You mean you observe the sun for military reasons?
No, the front line of science is where we come up against something no one has studied before. Where we can check which elements of commonly-accessible knowledge actually match our observations, and which elements need to be described more accurately. In order to start thinking, you need to look more closely. After many trials and errors, which take a lot of time, you may come up with the right idea. These days, states and institutions are engaged in the pursuit of science, but research work used to be an exclusive occupation. Only the elite, the most affluent people could afford to do it. Nicolaus Copernicus was supported by the Catholic Church, so he could spend his nights making observations and calculations. Johannes Hevelius owned a brewery, so he built telescopes out of passion and spent his time gazing at the sky. In those days, science was something pursued only by the economic aristocracy. Now, there is a whole system of support for scientific research. A certain percentage of people can devote themselves to working only as scientists. An arduous job, quite distant from the lustre of popular-science lectures. We sit and observe, we test hypotheses, and we try to come up with ideas.
We depend on the sun. These days, there’s probably no other piece of common scientific knowledge that doesn’t meet even with the slightest objection. The importance of the sun is certainly not in the same category as the Earth being spherical, vaccines being useful, or climate change being harmful. It’s absolute knowledge. There’s no escaping it. If there were no sun, we would not be here. But most people still don’t realize how powerful an object it is, in every respect. It’s a star.
A death star?
If we directed all of the sun’s energy at the Earth, the planet would evaporate completely in 11 days. Any decent-sized solar flare, which represents a fraction of the overall solar production, would instantly cause all the oceans on our planet to boil.
Where does such power come from?
The sun itself generates energy. Planets do so too, but on a completely different level. The difference is of tremendous magnitude. The sun produces energy through nuclear fusion, a powerful phenomenon that generates enormous amounts of energy. I’m not sure if we, as mankind, could ever consume so much. The sun’s surface temperature is 6000°C, which is pretty darned hot. But the inside of the sun reaches temperatures of well over 15,000,000°C, able to melt any matter. A stream of heat that could destroy everything; turn it into a ball of liquid.
The sun itself is no small ball.
Its diameter is 110 times greater than that of the Earth. It would take a million Earths to fill up the sun. We’re sufficiently far away from the sun to reduce its massive power to something we can cope with. Especially at our latitude, where we say it’s pleasantly warm on sunny days. Further south, however, people know that the sun can be a curse; its power can be destructive. The sun comprises practically all the matter in the entire Solar System, an overwhelming majority of it. All the things that move around the sun – all the planets, moons, planetoids, comets, and whatnot – all the orbiting matter is equal to a mere 0.2% of what is inside the sun. We’re just a tiny addition to this powerful object. The sun rules our part of the universe. We’re moving around the sun, but it will not let us out of its grip. Its mass is over 300,000 times greater than the mass of the Earth, so its gravitational field is correspondingly stronger.
The Earth means solid ground. You could land on the Moon, stand there, take a few steps. The sun doesn’t have this solid nature.
It consists of gas, which is ionized to form pure plasma in the inside and in the corona. Ordinary gases don’t react with a magnetic field, they ignore it. Plasma consists of a great many charged particles, which can sense a magnetic field very well. Plasma has to obey such a field, which is why some interesting things happen on the sun, such as the solar flares we have already mentioned. In the photosphere, which is the part visible to the naked eye and characterized by the lowest temperature, there’s partial ionization, but that’s also plasma.
We can see the photosphere. What is it that we can’t see?
When we look with the naked eye, what we can see is a rather thin layer in the sun’s structure. It is several hundred kilometres thick. From our perspective, the sun has sharp edges. But that’s an illusion, the star actually stretches much farther out. According to some definitions, we could even say that we’re actually living inside the sun – we’re part of what is called the heliosphere, or the area of the solar wind’s activity. Telescopes allow us to see only the sun’s external layers. When we include ultraviolet or X-ray observations, we can see that solar matter reaches much farther out. The sun is many times larger than what our eyes can see. The same holds true for the inside of the sun – we can’t see it through a telescope, either. That’s impossible, because the matter becomes opaque. We have to resort to certain other methods.
What methods?
For instance, we can conduct seismic studies of the structure of the sun, like we do on Earth. Of course, no one detonates explosives on the sun; we use the natural oscillations of the sphere. Basically, we analyse the sounds that pass through the sun. We can see the acoustic oscillations of the photosphere. When energy emerges from inside of a star, it’s not a calm flow. Many random things occur that constantly generate sound, meaning vibrations.
Does it get loud in there?
Yes indeed. A lot of things are happening there. We can record them using the techniques of spectroscopy. The central parts of the star are the most difficult ones to access. The more shallow ones are easier to study. The deeper we go, the harder it gets.
So it’s basically like hell? With particles, like damned souls, wandering around for millions of years before they reach the surface?
Yes, photons certainly don’t have it easy. The average photon, a carrier of the energy generated through fusion, has to wander through the sun for tens of thousands of years before it finally reaches the photosphere and nothing stops it from flying off into space. For sure, there are also photons that have been wandering inside of this star for millions of years, unable to get out, because it’s not so simple. We can compare this situation to a drunkard’s walk. Photons behave like a man who has consumed a lot of alcohol. He walks two steps and falls. He gets up immediately, but doesn’t know where he is, so he walks off in a random direction. He takes another two steps and falls again. Photons behave similarly, but they sometimes manage to get outside and escape the photosphere. There are also certain particles that fly out immediately, called neutrinos. They’re generated in fusion processes. They’re reluctant to interact with other forms of matter and simply pass through the sun. We’ve learned to detect them, but that’s not easy, because they can ignore the matter in the sun, so it’s easy for them to pass through our devices, too.
How do we catch them?
Right now, there are billions of neutrinos flying past us and through us. 100 billion neutrinos per second through each square centimetre. They are doing nothing to us, because they don’t see us. They ignore our form of matter. But they don’t do this perfectly, so we first managed to detect them in 1968. These observations are very difficult, they require huge detectors. The largest one is located on the South Pole and is distributed over an entire cubic kilometre in the glacial ice there.
Where are they flying to?
Off into space, roaming the universe. They only very rarely interact with the matter we know, but this allows us to detect them. We measure the stream of neutrinos coming directly from the sun, and this gives us information about the state of the sun’s core. We can estimate the conditions there, as the stream has certain properties that we can read. We can’t put a thermometer into the sun, so we figure out the temperature based on other processes. It took us many years to prove that neutrinos actually exist. A long time ago, scientists started saying that something might be wrong with certain reactions, that some particle we didn’t see could be getting generated. Several decades later, it was demonstrated experimentally that such a particle existed.
What did we know about the sun in the past, back when observations were made without advanced technologies?
From today’s perspective, we knew nothing.
Our ancestors prayed to the sun.
People prayed to the basic source of energy thanks to which we exist. Life on such a planet as Earth would be possible without harnessing the sun, but it would be much less developed, concentrated only around weak, terrestrial sources of energy. It is presumed that life on Earth may have first emerged around geothermal energy sources. After one or 1.5 billion years, photosynthesis appeared. Before that, life relied on the chemical processing of compounds dissolved in water. The easiest thing was to feed on iron compounds. When most of them were consumed, a certain hunger ensued: something new had to be found. Photosynthesis developed and transformed the Earth. Before that, there was no atmosphere like the one we want to protect now. For half of our planet’s lifetime, there was no oxygen in the atmosphere at all. If we travelled back in time and landed on the Earth back then, or if we travelled in space to a different planet at a similar stage of development, we would be unable to breathe. Humans evolved in the times when the sun had long served as the primary source of energy for life. Everything we eat, all the food we have, emerged thanks to solar energy.
How did photosynthesis originate?
As a result of evolution at the cellular level. We didn’t start studying the mechanisms behind that process until a decade or so ago. Before that, when we studied the history of life on Earth, we focused on fossils, which provide traces of advanced organisms, macroscopic ones. But the first four billion years involved evolution at the cellular level. No fossils were left behind by those processes. We now have advanced genetic tests at our disposal, so we can peer into the genes of single-celled organisms and gain insight into their evolution. We don’t realize that even at the level of a single cell, we are extremely complicated and evolved entities. Behind us, there are billions of years of constant change and struggles for survival.
When did the scientific study of the sun begin?
Galileo started to observe the sun using the first telescopes, which cost him considerable damage to his eyesight. He didn’t know that you can’t look directly at the sun, and so he tried to do just that. People had of course long known that the sun rose and set, that it was the main source of light and heat. In ancient times, some people argued that the sun was the most important object in our system. Ultimately, that was demonstrated scientifically by Copernicus.
But there are also earlier studies. For example, ancient Chinese records of sunspots.
Some groups of sunspots were and still are visible to the naked eye. Observations are not possible during the day, but we may be able to make them out at sunset, looking through a thick layer of the atmosphere. Something was noticed, but even in Galileo’s times, people could hardly see anything. Processes were seen, but they were interpreted differently. For example, it was suspected that large flocks of birds might be obstructing the view of the star. But thanks to observations and notes, we now have certain records of when large groups of sunspots appeared on the sun.
For instance, even from the Polish novelist Henryk Sienkiewicz. He expressed the belief that events on Earth and cosmic phenomena might somehow be closely linked.
That’s what happens when we know very little about something. We focus on what we can observe and how we might interpret it. It wasn’t until the 19th century that someone noticed that sunspots appeared cyclically. 100 years ago, we learned to measure the magnetic fields on the sun. It turned out that sunspots are regions of very strong magnetic fields. When the strength of the field grows, this inhibits convection, or the transfer of energy through the movement of matter [rather than through radiation – ed. note]. The transfer of heat from bottom to top is inhibited. It’s very difficult for the matter inside the star to move across the magnetic field lines, but it can move along these lines. Consequently, the photosphere gets darker, and we have a sunspot.
How long have we known that the chemical composition of the sun differs from that of the Earth?
For around 100 years. It was earlier believed that a star consisted of matter similar to its planets. Spectroscopic analyses of the sun proved otherwise. The sun is almost entirely made up of two elements: hydrogen and helium. Matter in the universe is fundamentally made of these two elements, everything else is just a slight admixture. Spectroscopy is a technique that allows us to determine the chemical composition of a star without touching it, from a safe distance. We study the spectrum of a star by taking its light and passing it through a prism or diffraction grating – devices that can segregate light out into wavelengths. What goes into our eyes every second is a mixture of different wavelengths. A prism refracts light of different wavelengths to a smaller or greater extent. We get a rainbow effect, or what the eye sees as a range of different colours, which are in fact different wavelengths. We can position a prism or a CD in such a way that we’ll see a rainbow. Using advanced devices, we can see more details, for example spectral lines, which are very narrow ranges of wavelengths where the amount of energy drops. These lines give us information about the matter emitting the light and the specific elements that comprise it. A vast majority of stars are made up of hydrogen and helium. Out in the Solar System, there are more heavy elements, so the composition of the Earth is different. But Jupiter and Saturn have practically the same chemical composition as a star.
Is helium created from hydrogen?
Hydrogen is constantly being converted into helium within the sun. However, a vast majority of the helium in the cosmos didn’t come from this stellar process. It was created in the first 10 minutes or so of the existence of the universe. The first stars were composed almost exclusively of hydrogen and helium. There was also some lithium, but it was quickly consumed, because it was a juicy target for thermonuclear reactions. Stars constantly generate heavier elements, and we’re ultimately made out of them. We’re made of star dust, there’s no question about that.
In a sense, we try to emulate stars. Some compare the processes occurring in the sun to a hydrogen bomb.
Bombs trigger a rapid conversion of all matter from hydrogen into heavier elements in a short period of time. This produces the effect of a colossal explosion. The sun doesn’t explode; it only swells and smoulders, as there are enormous amounts of matter that sustain fusion at an even pace. This generates the energy that lights up the whole of the Solar System.
Back in the 1950s, Professor Jan Mergentaler decided that the Astronomical Institute in Wrocław would start researching the sun. Our first coronagraph was built – a telescope that simulates the situation of a solar eclipse. Some pointed out that there were so many other objects that seemed more fascinating, but this research turned out to be extremely important for many reasons. If we look at other stars in our galaxy, 90% of them behave like our sun. That’s an important pattern. We can identify the phenomena occurring on the sun with a great degree of accuracy and then rescale them for other objects. It’s simply worth taking the best possible advantage of the star located close to us.
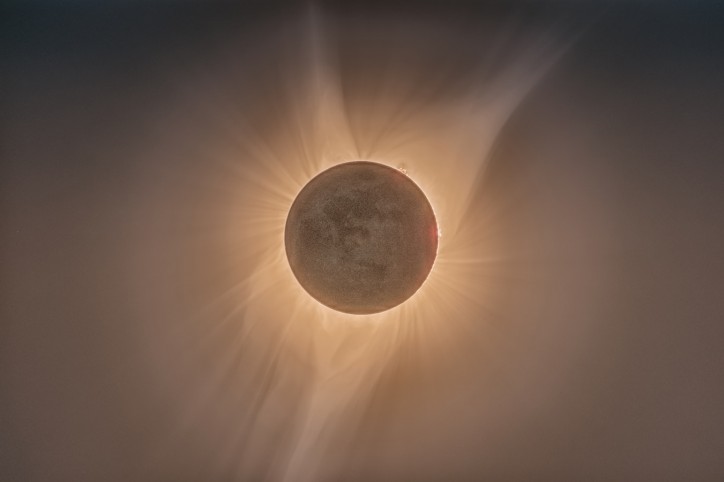
The Parker Solar Probe, launched last year, was able to approach the sun at a distance of six million kilometres. That’s a record-close approach, but it’s still an extremely long distance.
That’s a very bold mission, but it has been scaled back somewhat. According to initial plans, from the late 20th century, scientists intended to get within four million kilometres and reach where the solar wind is born and the sun’s atmosphere escapes freely into space. We’ve sent probes to various places, but usually in the opposite direction.
We’ll be travelling towards the sun again next year. This time, the European Space Agency will be sending a satellite called the Solar Orbiter.
It differs from the Parker probe in that it looks directly at the sun. The Parker probe ventured so close that if it had let even a tiny share of the light in, it would have been destroyed. So it looked sideways. The Orbiter will look directly at the sun, so it won’t get as close. We hope that we will get observations of better quality than those made from the Earth, if we’re flying three times closer. The probe has an advanced X-ray telescope, and we’re eager to see its observations. But the Orbiter must also cope with threats – an amount of energy that is 10 times greater than the energy that reaches us. At the moment of closest approach, the Parker probe received a stream of energy 600 times stronger than what we experience here. And that’s a lot, because we have one kilowatt per square metre on Earth. Hardly anyone knows how much that is, until they go to Greece or Egypt in the summer and try to sunbathe in full sun. Everyone hides under umbrellas. The Parker probe was 20 times closer to the sun, so it had to stay behind a protective barrier.
What did it observe? Was it possible to unlock what is probably the sun’s greatest mystery, namely why its surface is cooler than the more external layer, the corona, despite being closer to the source of energy inside the star? The Parker probe was expected to study that. That’s at least what NASA scientists looking after the mission claimed in official statements.
I’ll allow myself to be critical here. It could be difficult for the Parker probe to study this phenomenon. The hot corona is formed a lot closer to the sun’s surface than the probe could venture. We can’t say today which of the physical mechanisms heats up the corona. The suspects include magnetic waves. The probe’s magnetometers may find traces of this phenomenon, but if that proves impossible, we’ll at least rule out certain hypotheses.
So what is the Parker mission’s actual goal, as opposed to the one announced for publicity?
First of all, to check which of the versions of the solar wind generation model match observations best. The best situation is when we can reject a certain model. The data from Parker’s first orbit still keep coming in – we’re glad that the probe has survived in good health. That was the first approach; there will be a total of 24. The second orbit is scheduled for early April. In addition to the solar wind, the Parker probe also studies coronal mass ejections. The magnetic fields on the sun can become so contorted that they rapidly release energy. The effects include great bubbles of matter and magnetism, which are released from time to time. That’s what we call coronal mass ejections. When such a bubble reaches the Earth, it collides with our magnetic field. That causes a phenomenon referred to as a geomagnetic storm. It occurs two or three days after the bubble is released from the sun. In the late 1990s, it turned out that we’re at such a stage of civilizational development that a really strong geomagnetic storm could actually harm us.
In 2003, “Przekrój” wrote about that, about flares being responsible for fires and the explosion of gas pipelines, power grid disruptions on large areas, cancelled flights, ATM failures.
That still holds true. In 1989, there was a famous blackout in Canada. Power lines were burned out in Quebec and in the north-eastern part of the United States. The Earth’s disrupted magnetic field induced chaotic currents in a way that made transformers break down. Other elements of the grid became overloaded, and a domino effect began. That was a serious lesson. We know from the analyses of historical observations that it was not the most severe geomagnetic storm experienced by our planet. There were two very powerful ones known before then: one in 1856 and the other in 1922. Back then, they did no damage, because there were no power grids. At mankind’s current level of development, we need to construct our power grids with dangers from the sun in mind. The same holds true for our gas pipelines. Several hundred kilometres of a metal pipeline with something being induced in it by the Earth’s magnetic field – I’d rather not imagine that, I don’t want to be anywhere near it. That’s why practically all new gas installations are not made of metal. That’s a very reasonable idea. However, power lines have to be made of conductors. If a geomagnetic storm occurs in the middle of a hot summer, our air conditioning will break down and we’ll revert back to nature. By causing sudden voltage surge impulses, geomagnetic storms will act like Magneto from the X-Men. That’s a somewhat exaggerated picture, but the sun could, roughly speaking, work in a similar way. That’s why over the past two decades more money has been spent on studying what is called space weather.
Are the electricity providers in Poland up to speed in this respect?
No, not really, as far as I know. In Wrocław, we are the main solar research centre in Poland. No one has asked us about that. Of course, there are international information agencies – after all, studies of the sun are not classified. But you would have to ask electricity providers if they make use of them. They don’t talk to us.
I’ll ask them, then. What are the forecasts?
It’s very difficult to predict accurately what will happen on the sun. It is characterized by chaotic processes. That’s a problem for astronauts, who must be informed on an ongoing basis about potential solar behaviour. After all, they sometimes leave the station. Sometimes, just to be on the safe side, a statement needs to be issued: “This week, don’t leave the station, because we can’t rule out potential flares. No one can take a spacewalk until the observed group of sunspots goes away.” Otherwise astronauts would get an additional dose of radiation over their whole body. The biggest geomagnetic storms occur not when the star’s activity is at its maximum, but when the sun’s activity subsides. That’s when there’s a risk of the strongest flares and ejections. Now we are at a minimum, and we’re waiting for a new cycle. The first two of its spots have been detected at our institute. It’s hard to say when the new cycle will start. Maybe next year.
How long is one cycle?
Cycle lengths vary from nine to 14 years, with 11 years being the average. Today, we know that we can’t predict what the next cycle will be like. This was demonstrated by the previous cycle, which proved to be distinctly weaker than the previous ones.
Back when we got our news from newspapers, we could live more peaceful lives. They would not go up in flames as a result of geomagnetic storms triggered by the sun’s activity. But I don’t know what will happen to our TV sets and computers. Fortunately, the internet is isolated, because it uses optical fibres.
Will a geomagnetic storm not destroy our data out in the cloud?
Data clouds are protected. They’re not single copies. There are whole server farms with disks. Files have many different copies. However, I can imagine a situation in which major overvoltage in the grid causes damage to such server farms.
Should we expect more frequent geomagnetic storms?
We can’t predict that. We simply have to keep observing the sun on an ongoing basis.
Parts of this interview have been edited and condensed for clarity and brevity.
Dr Paweł Preś:
A heliophysicist employed at the Astronomical Institute, University of Wrocław. He observes not only the sun but also other similar stars that behave analogously, in particular their flares. He is an enthusiastic popularizer of science and co-runs the Planetarium Lab at the Astronomical Institute, University of Wrocław.
Translated by Daniel J. Sax