
Not only does the immune system have to fight intruders but it must also take care not to damage its friends. As the teacher in the school for white blood cells says in the series Once Upon a Time… Life: “It is important that you know who to attack and never make a mistake.”
Anthills are fascinating. An ant colony is like a single organism, whose cells (individual ants) make up the organs (castes of ants), fulfilling different functions: defence, food supply, reproduction. Ants fiercely defend access to their colonies, knowing how valuable the resources inside are for other animals: defenceless larvae, the worker ants that feed them, and food stores. The latter, for some species of ant, such as the honeypot ant (Prenolepsis nitens), come in the form of living larders of defenceless worker ants, stuffed full with food right up to the holes in their chitin armour, stuck immobile in one place…
Worker ants, and in particular soldiers, recognize most of their intruders by smell and fight; but not all. For example, the larvae of a beautiful butterfly, the Scarce Large Blue, have learned to outsmart the ants, secreting pheromones similar to those of ant larvae. This guarantees them free food, (they are fed by the worker ants looking after their own larvae) and security (thanks to the very same defence system they were able to fool).
Each one of us, as multicellular organisms, is precisely such a colony. And the defenceless, specialized cells inside our bodies are a risk-worthy target for viruses, bacteria and all types of parasites.
The need to have a defence against other organisms and viruses is probably as old as life on Earth itself. Even bacteria have a refined defence mechanism; they don’t just fight an infection when it appears, but they also manage to remember individual varieties of viruses in order to deal with them more effectively in the future. No wonder then, that all multi-cellular organisms evolved immune systems – specialist systems of cells responsible for defending our ‘colony’ from any enemy attacking us from within.
A computer made of cells
When a disease-causing bacterium gets into our body, it sets off a whole cascade of complex processes. The immune system (depending on how you count it) contains several dozen different types of cells that work together and communicate either directly (through contact) or via signals; special molecules. Our immune system is no less complex than our nervous system! Cells such as microphages or dendritic cells recognize the intruder, absorb it and notify other parts of the system. A complementary system of molecules found in the blood stream are activated and perforate the bacterial cell membrane like machine gun bullets. Water gets in through the holes and this bursts the microbe like dynamite. Other divisions of different immune cells appear at the site of the infection, summoned by the signal molecules released. White blood cells and Tc leukocytes – known in English at killer T cells – arrive. However, they don’t kill the bacteria or viruses, but identify the infected cells from our own body and press the self-destruct button; a mechanism known as apoptosis. NK cells – from the English, ‘natural killers’ – work in a similar fashion. Upon encountering bacteria, neutrophils (another type of white blood cell) are also able of committing hara-kiri (ritual suicide by self-disembowelment) – they fall apart, spewing out their contents, full of bactericidal substances and strands of DNA which, armed with sticky molecules, catch the bacteria in their net.
And this is only a tiny fraction of what’s happening. I don’t want to bore you with the fine details of the immune system, but simply show you its complexity and richness. Yet why is it, in fact, so complicated?
Above all, our immune system has to distinguish between friend and foe. In the end, the body is full of all sorts of cells. This is an extremely difficult task because an enemy often tries to disguise itself (like Scarce Large Blue larvae in an ant colony), or even hide inside our cells (like a virus or some bacteria). Our own cells can also become our enemies, when they become unstable due to a mutation and start to replicate uncontrollably, as with cancer cells. However, on the surface of the skin and inside the intestines, hugely complicated ecosystems are at work, made up of bacteria that we need in order to function properly and that should not come under attack from our immune system. In essence, we live in symbiosis with them.
Defended by Lego blocks
The first specialist immune cells, known from many simple organisms, are similar to human microphages – they travel round the body eating anything that seems suspicious. In the excellent series Once Upon a Time… Life, the macrophages are depicted as large, frog-like vehicles gobbling up bad bacteria. Microphages react directly to different substances that are secreted by bacteria or found on their surfaces. This works really very well, as proven by the existence of so many organisms and also by the fact that this setup is one of the two pillars of our immune system; we call it a “non-specific immune response”. Macrophages, the complementary system and ‘natural killers’ all belong to this system, and these cells usually have short but turbulent lives.
However, the non-specific response has two fundamental flaws: first, it must be fully encoded in DNA, and the number of genes that can be found in DNA is limited. Second, it cannot learn. If a bacterium is not in the catalogue – our DNA, fixed at birth – it won’t be recognized. More or less half a billion years ago, at a time when the first fish evolved, a new defence mechanism appeared that was free of these flaws: the adaptive immune system (in the series Once Upon a Time… Life, portrayed as hovercraft, whose pilots are first sent off to school).
The key to the new system was antibodies – immunoglobulins, which in a very precise manner are able to recognize specific proteins on the surface of bacteria or cells infected by viruses. Small changes in the structure of a protein can determine whether a given antibody will recognise E. coli, a flu virus or gonorrhoea. But there are a lot of microbes that our body must learn to recognize. So, where do we get the thousands (or hundreds of thousands) of immunoglobulins from if our DNA only codes for 20,000 genes?
Any child who has played with Lego blocks during their childhood can answer this question. Antibodies are comprised of segments and each segment has several dozen different variants (like different coloured Lego blocks), which (also like Lego) can be put together in different ways. It is estimated that there are thousands of billions of different possible combinations; in fact, an inexhaustible pool of possibilities.
In truth, it was not vertebrates who discovered how to construct immunoglobulin variants. Insects, for example, also do it, although less effectively. Snails have a similar system, although based on a different group of proteins than those of immunoglobulins, and probably many other types of multicellular organisms can also do so. The key to this invention was something else. The cells of the adaptive immune system, the T and B leukocytes, randomly modify their own DNA, creating new combinations from these blocks. In this way, every newly-created B or T cell has slightly different DNA to the rest of the organism.
Most of the combinations will be useless, but some of the cells by chance will carry antibodies that can recognize foreign bacteria. When a cell with an effective variant of antibody meets a bacterium, it starts to divide, passing on its combination to its daughter cells. Some of these are memory cells; a living catalogue of antibodies that can recognize an enemy. This is how an organism can perpetuate working combinations. Thanks to memory cells, when the same strain of bacteria or virus infects our body again, the immune response will be much faster. This is why we don’t get ill twice from mumps or measles.
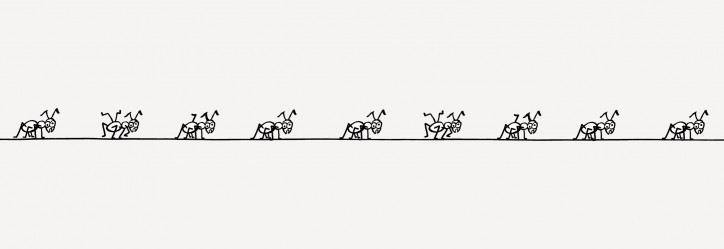
No jaws, but lymphocytes
Lamprey and hagfish are nightmarish looking fish (unsurprisingly, they are used in horror films). In fact, from the scientific perspective, they are not even fish, but vertebrates from the Cambrian period (over 500 million years ago); living fossils. Although on the outside they look a bit like eels, they have no jaw; hence their scientific name – agnatha (from the Greek, meaning ‘no-jaw’). This is most probably what our ancestors looked like; agnathas were the first vertebrates. Since the time when the protoplasts of contemporary fish and all other vertebrates appeared (in the Ordovician era, several dozen million years after the Cambrian), agnathas evolved in parallel, independently of other vertebrates.
The non-specific, untrainable immune system of lampreys and hagfish is almost identical to that of other fish and quite similar to that of humans. Just like a goldfish or a human, a lamprey has macrophages in its body that hunt bacteria. However, a dozen years ago or so, a fascinating discovery was made. It turns out that agnathas also have an adaptive immune system and, although this works on the same principle, its construction is completely different. The agnatha body has white blood cells, similar to our T and B lymphocytes, but hagfish and lamprey don’t have immunoglobulin, which could be used to identify pathogens. In order to create the numerous combinations to work as antibodies, they use a completely different type of protein. One can see, therefore, that although in part we have a common evolutionary heritage – the non-specific immune response and white blood cells – the agnathas made their discovery independently from the remaining vertebrates. This is an example of convergent evolution, just as with the independent development of the ability to fly in bird-like dinosaurs, mammals such as bats, and insects.
How did it happen that, in such a short time from the evolutionary perspective, two related groups of organisms created different, yet similarly functioning immune mechanisms? Certainly, the creation of an adaptive immune system made possible the next step in evolution: the appearance of long-lived lymphocytes that are able to replicate. Although invertebrates have cells that look like macrophages, none of them, with one exception, have anything even remotely resembling lymphocytes.
But this doesn’t answer the question as to why vertebrates needed an immune system able to learn.
One hypothesis explains the appearance of a complex immune system through a symbiosis with bacteria. In a situation where the body has to learn to distinguish between thousands of necessary and thousands of dangerous micro-organisms, and, in addition, where the ecosystems of these micro-organisms can change, an immune system with rigidly-coded DNA is not enough. The aforementioned exception could be the confirmation of this hypothesis. Apart from vertebrates, echinoderms such as starfish and sea urchins also have lymphocyte-like cells. These also often live in symbiosis with bacteria.
The bacteria found in the digestive system are not just commensal organisms, i.e. stowaways tolerated by our bodies. Not a bit! We need them to live: they digest for us the food that we cannot digest for ourselves (e.g. some types of roughage); they produce the vitamins that we need and, moreover, they protect us from other disease-causing micro-organisms, even creating their own antibiotics. Therefore, we live in symbiosis with our bacteria. But it takes two for symbiosis and both sides must learn to live with each other – which means, among other things, effective communication.
In reality, over the course of evolution, numerous channels of communication were created between bacteria and our immune system. In the intestines, where thousands of bacteria species live, there are also masses of immune system cells that learn to tolerate ‘good’ bacteria and the substances that comprise the natural ingredients in our food. Unfortunately, our contemporary lifestyles, in particular the excessive or unnecessary use of antibiotics, can badly disrupt this delicate communication network. Many illnesses are related to disruption of the microbiological balance: non-specific gut inflammation, various allergies and even obesity.
Of mice and men
The riddle of the evolution of the immune system in animals is not nearly so theoretical as one might think, but has measurable consequences for our health! Research into human diseases, new medicines and vaccinations is, to a great extent, conducted on ‘organism models’ of animals: mice, rats, ferrets and even fish. However, the common ancestor of mice and people lived tens of millions of years ago and, since then, our immune systems have evolved independently. How much can studies on mice tell us about the mechanisms of human diseases?
Several years ago, an article appeared in Proceedings of the National Academy of Sciences, entitled “Genomic responses in mouse models poorly mimic human inflammatory diseases”. A year later, in the same publication, a text appeared with an identical title, but the word ‘poorly’ had been replaced by the word ‘greatly’. On top of this, both papers were based on the same research data! The apparent contradiction is down to evolution. Certain elements of our immune system are very old and are common to both species, while other elements differ. Our task is to identify which parts are the same and which are different. A little later, together with my colleague, Teresa Domaszewska, we published the results of a test like this. Initially we even wanted to use the same title, only with the word ‘partly’, in line with evolutionary logic.
How can we help?
Television and the internet are full of advertisements for substances that are supposed to be miracle boosters for our immunity. Considering evolution, this seems, at the very least, rather strange – if our immune system can work so much better after drinking echinacea tea, then why hasn’t evolution been able to strengthen it over millions of years?
The answer to this question is simpler than it might appear: echinacea tea, although it may be tasty and improve our mood, doesn’t actually improve our immunity: numerous clinical studies have been carried out on the effectiveness of various echinacea preparations for treating colds and, unfortunately, there is no convincing evidence to support their effectiveness. And a good thing, too, because, for as long as we are in good health and eat properly, there is no need to stimulate our immunity further; there are even good reasons to avoid it. An over-zealous immune system causes auto-immune diseases and allergies. So, if tea (or any other concoction) of echinacea really ‘worked’, as some advertisements suggest, we should treat them as a dangerous medicine only to be used in certain circumstances.
Unfortunately, as is often the case, although it is difficult to help, it is all too easy to cause damage. Lack of sleep, substances like alcohol, or vitamin D deficiency all significantly weaken our immunity. The good news is that, in order to strengthen an immune system weakened by bad habits, one just has to stop those habits. The bad news is that we can’t just buy regular sleep and better food for a handful of change in a pharmacy. The only exception to this is maybe vitamin D, but it is worth talking to a doctor about this (the correct dosage depends upon age, weight, gender and confirmation of a deficiency).
But maybe there is another way to take advantage of the natural skills of our bodies to defend ourselves from infection? The adaptive immune system can learn and can fight much more effectively against pathogens that it has already encountered; therefore, can we not teach it to react to danger without the need to go through the disease for the first time? It is easy to imagine that dead bacteria and viruses (or their fragments) would be sufficient in order to train one’s immune system; to prepare it for real danger. In this way we can save ourselves from illnesses and from having to taking medicines, including antibiotics that can ruin our alliance with our gut bacteria. It turns out that, in fact, this method works fantastically, and has been doing so for more than 200 years. These are protective vaccinations.
Healthy as a rule:
Eat everything that is green.
Drink water with lemon first thing.
Snack on almonds and berries.
Go for a walk!
Say goodbye to sugar.
Give up processed food.
Don’t avoid vegetables and porridge.
Limit the amount of meat you eat.
Smile as often as possible!
MK
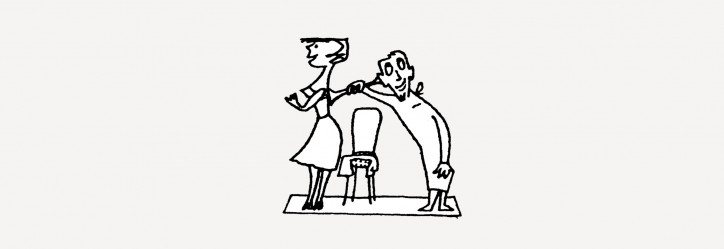
Translated from the Polish by Annie Jaroszewicz